What exactly causes a plant to flower? We do not yet have a complete answer to that question, but we do know a great deal about the mechanisms that trigger the response. There is no single phenomenon that causes flowering, nor is there one magical hormone that is responsible for it. Plants flower in response to several triggers that lead to a fairly complex chain of physiological and genetic responses, which ultimately cause a change in the morphological characteristics of the flowering apical shoots. Chief among these triggers are an effect of the light known as photoperiodism.
Photoperiodism means the plant’s response to certain light signals, including both the duration and the quality of the light it receives. Plants do not sense light in the same manner as people or animals sense light. In plants, the part of the electromagnetic spectrum which we perceive as light acts by providing energy for specific photo-chemical reactions in both control and energy production pathways. Animals also use light energy to ‘see’ the world around them. Light is a duality, existing both as a discrete particle (a photon) and as a wave. The higher the frequency (shorter wavelength), the higher the energy state of the quantum bundle known as a photon (see figure 1). The photo-chemical systems within plants are designed to capture specific frequencies of light and harness its energy to perform chemical reactions.

Colours of the spectrum
Plants capture light energy for two basic reasons: to make carbohydrates, and to control some of the thousands of processes that occur in the plant cells. Here, we are only interested in process control, but the wavelengths used to make carbohydrates are roughly similar. There are basically four colours oth the spectrum that plants work with:
- UV (ultraviolet) from 340 – 400 nanometre
- Blue from 400 – 500 nm
- Red from 600 – 700 nm
- Far red (the start of infra-red) from 700 – 800 nm
Light collection points
These figures are not absolute because actually the colours overlap and a plant will use some of the energy from 500 – 600 nm too, although not much. The plant makes use of different pigments to capture different wavelengths of energy. Broadly speaking, the four bands of electromagnetic energy control the activities of the plant through three collection points, or light absorbing pigments;
- cryptochromes (blue and UV)
- phytochromes (red and far red)
- phototropins (blue and UV)
The light collection points act like switches that turn on and off certain processes in the plant, and regulate others. While a human will only perceive the colours (wavelengths or frequency) reflected back to them and experience only a brightening or a dimming of the light level, plants are also sensitive to the light shift among frequencies that show as intensity to us.
Plants grown in the shadow of others receive much more red and far red light than they do blue light. They are sensitive to the shift from red to blue light that occurs naturally at sunrise, and the opposite shift that occurs at sunset. They are also sensitive to changes in the time when these daily events occur. The different pigments act as switches that are triggered by the energy of a specific wavelength as a ratio of one frequency to another. Even the absence of light affects a plant’s response through these control centres. All these controls affect the process known as flowering.
Light controls the natural rhythms of the plant (as it controls – for example – the sleep patterns of animals too!). These natural rhythms, or Circadian Rhythms, are inherent in all life forms. Life has a series of events it goes through during the course of each day. There are periods of activity and periods of rest. There are times when fuel is consumed and other times when certain activities or tasks are performed. All of these activities become programmed into a more-or-less 24 hour period.
It is inefficient to produce the chemicals used for capturing photons when it is dark (although some are). Just like a factory, components need to arrive when needed, stock taking must be done and a minimum level should be kept available, and assembly lines should roll when all the right parts are there. Light determines these rhythms, and not only through its presence but its quality as well.
A plant senses both the quality and the quantity of the light it receives. Based on environmental factors such as air quality or the time of year, the plant will sense a different ratio of colours. This difference is basically measured by the pigments which, when coupled with other triggers and processes, control what the plant ‘does’, and when. It sets the biological clock in the plant so that all the plant’s processes continue to run in harmony.
Cryptochromes sense the direction of the light and its quantity. Responses governed by cryptochromes include;
- stomatal function,
- gene transcription and activation,
- the inhibition of stem elongation,
- pigment synthesis,
- and the tracking of the sun by the leaves.
Phototropins, the other blue light receptors, are responsible for phototropism or plant movement, and the movement of the chloroplasts inside the cell in response to the quantity of light as a damage avoidance system. There is also some evidence that they activate the guard cells at the opening of the stomates.
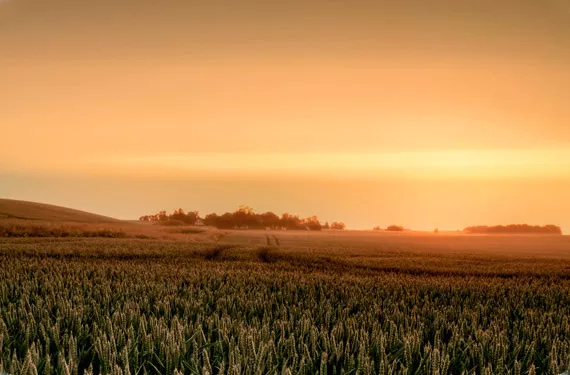
Phytochrome: Pr and Pfr
Phytochrome is a complex of pigments that occurs in two basic kinds:
- one that responds to red light (Pr)
- and another that responds to far red light (Pfr)
Depending on the light frequencies that they absorb the most (even though the other frequency will also activate it and blue light too). The two pigments generally convert back and forth, with Pr converting to Pfr with red light and vice versa (although some forms of Pr/Pfr lose the ability to reconvert depending on the amount of light, the intensity, or the quality of the light received). The active form, which triggers responses such as flowering, is Pfr. Red light exerts the biggest influence on photomorphogenesis (the effect of light on plant development) and far red light can sometimes reverse Pfr responses.
Phytochrome controls many functions such as
- gene expression and repression
- gene transcription
- the elongation of seedlings and stems
- germination
- photoperiodism (the flowering response)
- shade avoidance and adjustment to differing light levels
- chlorophyll synthesis.
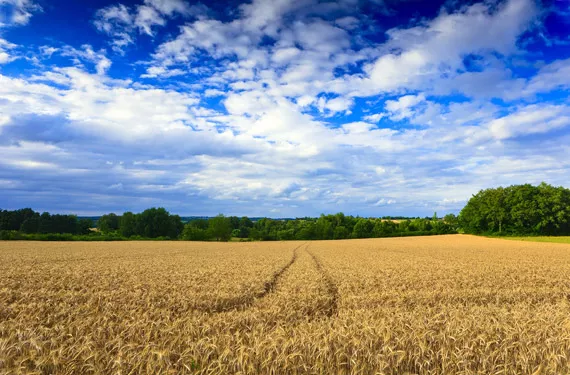
One example of a red light response is the change in the light interval from long days to short days, which will trigger flowering in short-day plants. This is because the plant senses the change through the ratio difference between red light and far red (or no light), and begins to change its physiology from a state of vegetative growth to floral growth. While the plant is receiving light, the ratio of Pr to Pfr (Pr: Pfr) is approximately in equilibrium (in fact, Pfr is slightly higher). Pr is converted to Pfr by red light and Pfr is converted back to Pr by far red light. As the sun sets, the amount of far red light exceeds the amount of red light and the levels of Pr increase, resulting in a slightly higher concentration of Pfr and a lower concentration of Pr.
Pr is produced naturally by the plant during the darkness and accumulates. Pfr also slowly breaks down to Pr (its half-life is approximately 2.5 hours). The next morning, there is total light again and the ratio of Pr to Pfr returns to equilibrium. In this case, it could be said that Pfr is like the grains of sand in an hour glass. It is currently thought that when Pfr concentrations are low and Pr is high, short-day plants flower and long- day plants do not. When Pfr concentrations are higher and Pr concentrations are lower, long-day plants flower and short-day plants do not.
Long-day and short-day
If we take two plants, one which is set to flower at a day length of 10 hours light/14 hours darkness (a short-day plant) and the other set to flower at 14 hours light/10 hours darkness (a long-day plant), the period that determines flowering is actually the night. This process is illustrated in figure 3. In effect, the short-day plant needs 14 hours of darkness to accumulate Pr and convert enough Pfr to Pr for the level of Pfr to be suppressed for long enough overnight for a morphological change to begin. This change becomes irreversible after a certain number of days. In a long-day plant, this process is basically the same but reversed. They respond to the presence of higher levels of Pfr.
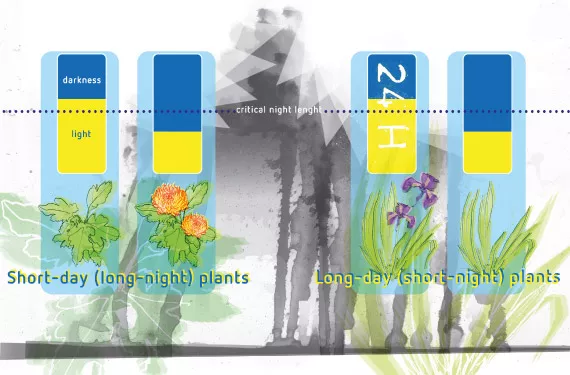
The length of time for which Pfr is the predominant phytochrome is what causes the plant to begin flowering. However, if the Circadian Rhythms are not right, and initially they will not be, the components needed to effect change may not be present at the beginning and the rhythms will have to ‘catch up’ before the change begins. Pfr ceases the repression of Florigen, the flowering signal, or it stimulates expression, and the signal makes the plant flower. Basically, the levels of Pfr tell the plant how long the night is.
Florigen, the flowering signal
Florigen, once described as a theoretical hormone, is now generally described as messenger RNA known as FT mRNA. In very simple terms, this is a protein molecule that is produced on a portion of the DNA of a plant in an area known as the FLOWERING LOCUS (T). This protein is like a key which searches out a specific lock that it will fit into. When the lock is turned, this initiates other processes. When combined with another gene known as CONSTANS (CO), it is now generally accepted that this begins the change from vegetative to flowering states. So the change to flowering by a plant involves external signals which affect, control and run the processes of the plant and trigger gene expression. All of this is triggered by the changes in the light which are picked up by the plant.
Flower response
There are basically five types of flower response in plants.
- There are short-day plants (SDP), which simply require a shift to short days and long nights in order to flower.
- There are long-day plants (LDP), which require the opposite.
- Then there are long-day short-day plants (LSDP)
- And short-day long-day plants that require a specific amount of time as a long or short-day plant followed by a short day or long day to flower.
- Finally, there are day-neutral plants (DNP), which require the same light functions but flower on triggers other than day length.
In all cases, it is not only the type or quality of the light received which triggers flowering, it is also the duration of the light (in all cases but the DNPs).
To be precise, it is the duration of the absence of light at night which triggers flowering, but based on processes and metabolites (Pfr, etc.) that the light has driven. It is important to understand that there are thought to be many other processes which play a role along with those described here, including the interaction of other genes and hormones such as GA (gibberellic acid).
Light is critical for all life, but especially plant life, where it not only produces the substrates for growth and metabolism, but it also establishes the rhythms and cycles of daily routines. Light controls critical aspects of survival and propagation; it sets the tempo for life in all organisms. Just as importantly, not all light is equal as far as a plant is concerned. The correct ratios of light (blue to red, red to far red, and so forth) have to be available for the plant to function correctly. Just like everything else, a plant can get too much of a good thing. In the end, however, while light is absolutely critical to plants, it is only a part of the overall equation of life.
Bibliography
- Aushulz. "file:Chlorophyl_ab_spectra2.png." Wikipedia. http://en.wikipedia.org/wiki (accessed 4 10, 2011).
- Huang, T., Bōhlenius, H., Eriksson, S., Parcy, F., Nilsson, O. "The mRNA of the gene FT moves from leaf to shoot apex and induces flowering." Science 309 (2005): 1694-1696.
- Kasperbauer, M. J., and D. L. Karlen. "Plant Spacing and Reflected Far-Red Light Effects on Phytochrome-Regulated Photosynthate Allocation in Corn Seedlings." Crop Sci. 34 (1994): 1564-1569.
- Koning, Ross E. Photoperiodism. 1994. http://plantphys.info/plant_physiology/photoperiodism.shtml (accessed May 31, 2011).
- Taiz, L., and E. Zeiger. Plant Physiology. 3rd. Sunderland: Sinauer Associates, Inc., 2002.
- Zeevart, Jan A.D. "The Plant Cell 18:1783-1789 (2006)." The Plant Cell. August 2006. http://www.plantcell.org/content/18/8/1783.full#BIB18 (accessed June 9, 2011).